Time to read: 7 min
Historically, gear fabrication methods have been classified into three main categories: generation, forming, and form cutting. As new gear manufacturing technologies are developed, new methods will be added to the list, but for now, those three are still the most common ways for machining a gear:
- Gear generation uses cutting tools in the shape of the desired gear profile to create the gear (i.e. rack cutters, gear shaping, and gear hobbing)
- Gear form cutting involves tools that are used to create the gear profile (i.e. gear milling, shaping, slotting, planing, and EDM)
- Gear forming creates gears without using cutting tools (i.e. rolling, casting, powder metallurgy, 3D printing)
Gear Generation
Sunderland Method
Rack-type cutters are one of the main gear manufacturing methods. The gear rack cutting process is also known as the Sunderland Method or the Sunderland System. This method utilizes a gear machine which consists of a rack cutter with rake and clearance angles that creates the teeth profile on a gear blank. It uses the specific relative motion between the workpiece and the cutter during machining to produce teeth profiles and is similar to a rack and pinion, which is depicted below.
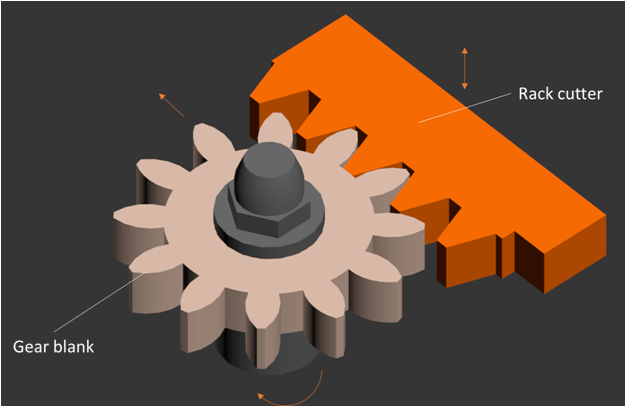
The teeth profiles follow a geometry that consists of an involute of a circle — basically, a spiraling curve traced by the end of an imaginary string unwinding itself from that stationary circle — or if you trace the point of contact from one tooth to another as shown in the following image.
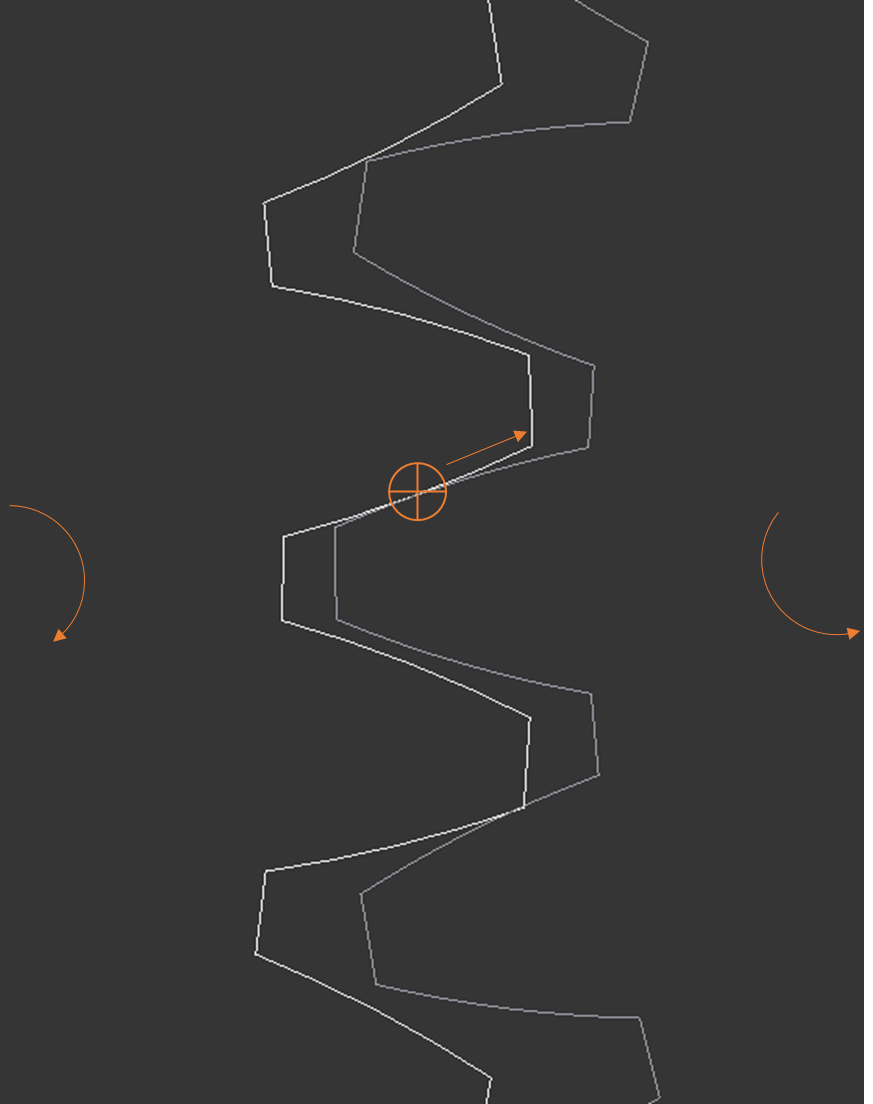
The Sunderland Method is excellent at creating teeth of uniform shape, and all gears that are cut by the same cutter will, in theory, gear correctly with one another. So, gear designs that require high precision — even double helix gears — can be fabricated with this method. The Sunderland Method is also versatile and cost-effective, especially for medium to high-volume production runs. And because the Sunderland system has been actively maintained since its invention, manuals and documentation are widely available and relevant even for new machine designs.
Gear Shaping
In this method of gear generation, a cutter and gear blank are connected by gears so that they don’t roll together as the cutter reciprocates. The cutter starts carving its way to the desired depth, as seen in Figure 3, then the cutter and gear blank rotates slowly as the gear teeth are cut.
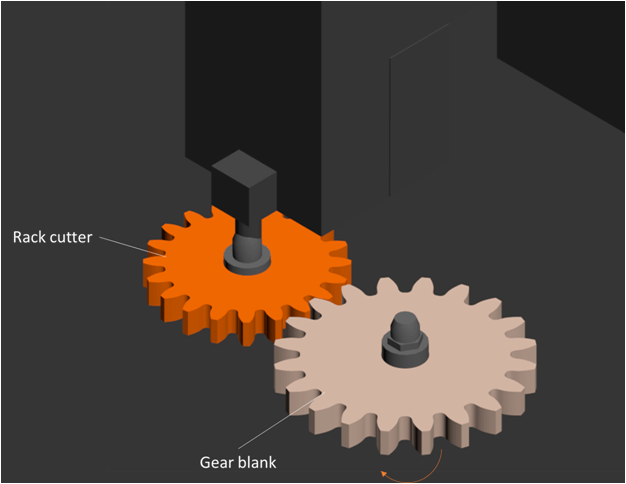
Gear shaping is commonly used for cutting spur gears, herringbone gears, and ratchet gears. This method can be used for other types of gears, but since it uses a cutter that reciprocates the gear shape, it’s often utilized for the gear types mentioned above. Since there isn’t a complex programming requirement (it’s just cutting the same as the rack cutter has into the gear blank), the setup during production is simpler. You can still manufacture these types of gears with other methods, but gear shaping provides speed, design, and setup advantages during mass production. Gear shaping is not the best option for internal gears and worm gears, due to the position of the cutter (on the outside) and cut direction.
Gear Hobbing
Gear hobbing produces gear teeth by rotating a cylindrically shaped cutter called a “hob” (hence the term gear hobbing) depicted in the gear hobbing machine interface below. The hob can be single-threaded, depending on how many teeth per revolution should be generated.
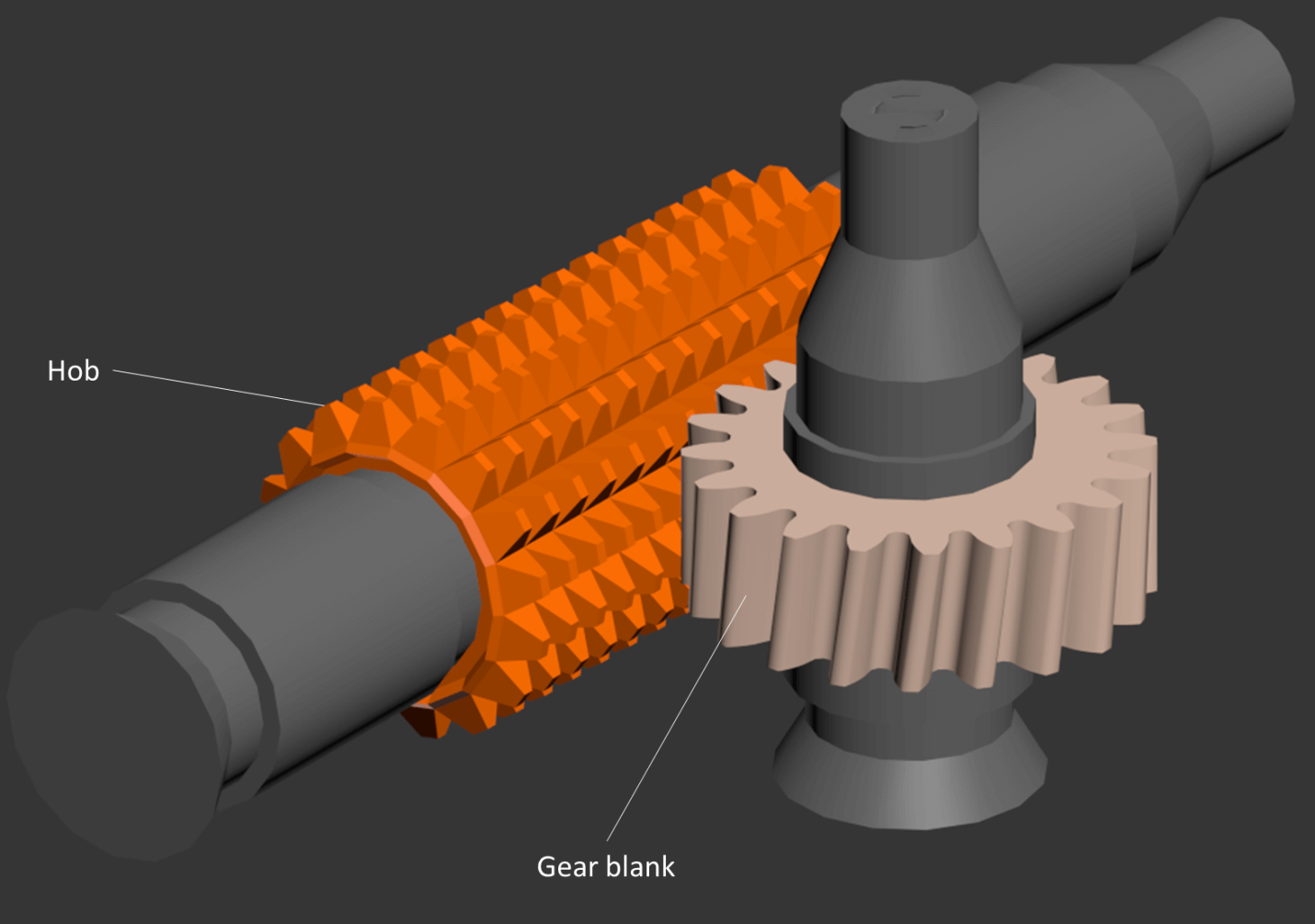
Spur gears are most often fabricated with this method, although a variety of other gears — like cycloid gears, helical gears, worm gears, ratchets and sprockets — are all made by hobbing. A well-designed hob is critical, especially when cutting a complex geometry, and this process typically does not work for internal gears. Similar to gear shaping, hobbing has setup advantages, but only for exterior cuts since the hob is cutting the outside of the gear blanks.
Gear Form Cutting
Form cutting generally isn’t preferred because of its limitations — low productivity and poor quality. However, the various form-cutting techniques are useful alternatives for repair and maintenance when necessary.
Shaping, Planing, and Slotting
When shaping, the workpiece is fixed and the tool on the ram is moved back and forth across the workpiece.
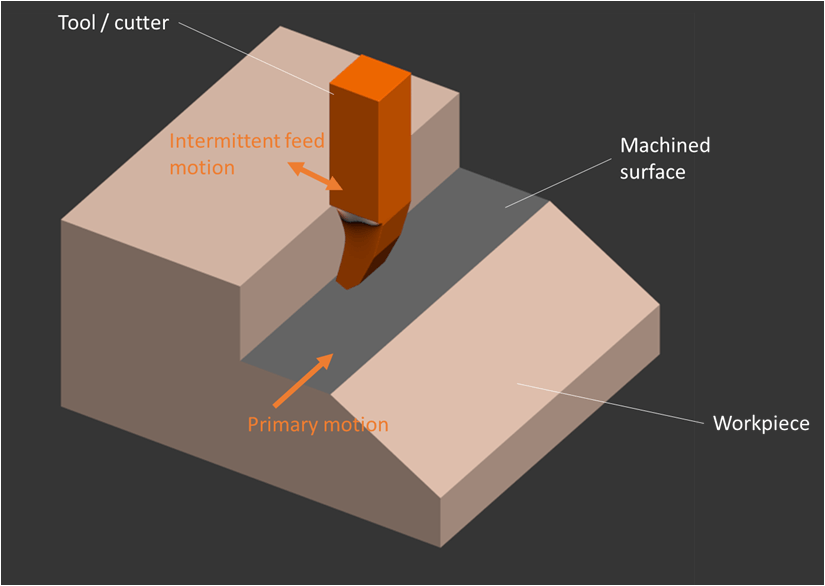
When planing, the tool is fixed and the workpiece travels on the table back and forth under the tool.
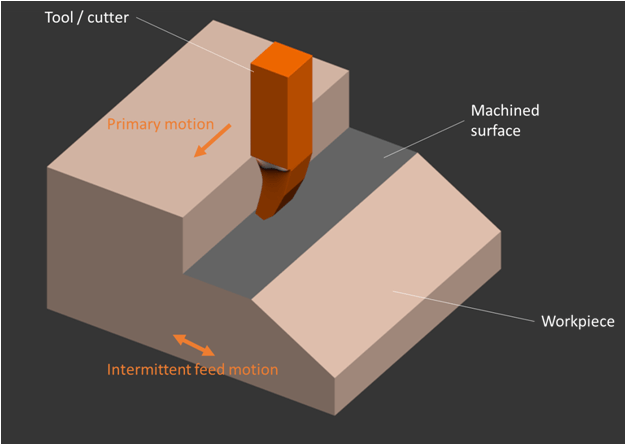
When slotting, the workpiece is held stationary and the tool on the ram is moved up and down across the workpiece.
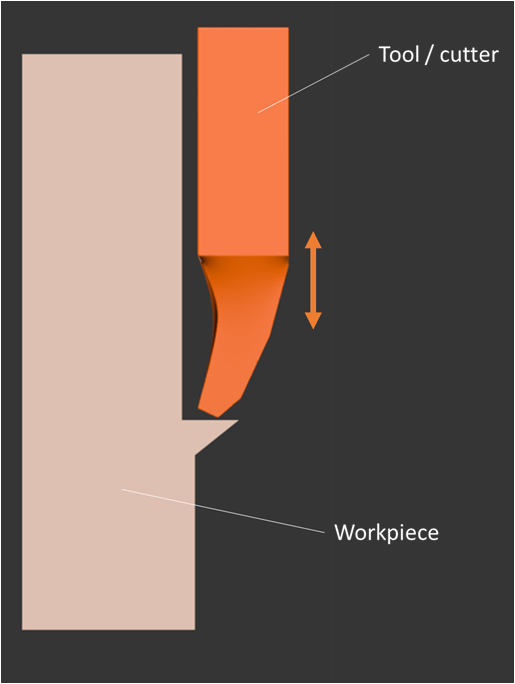
Both shaper and planer gear machining tools cut in straight lines. However, the shaper handles small-size geometries, while the planer is built for larger applications. Shapers can be used to create slots, grooves, and keyways. Slotting is essentially a vertical shaper that cuts internal gears and grooves.
Milling
The milling form cutting method is relatively limited in its use but is well-suited to create complex gear geometries. CNC milling is utilized to machine helical and spur gear wheels for various industries, including automobile transmissions, and hob cutters.
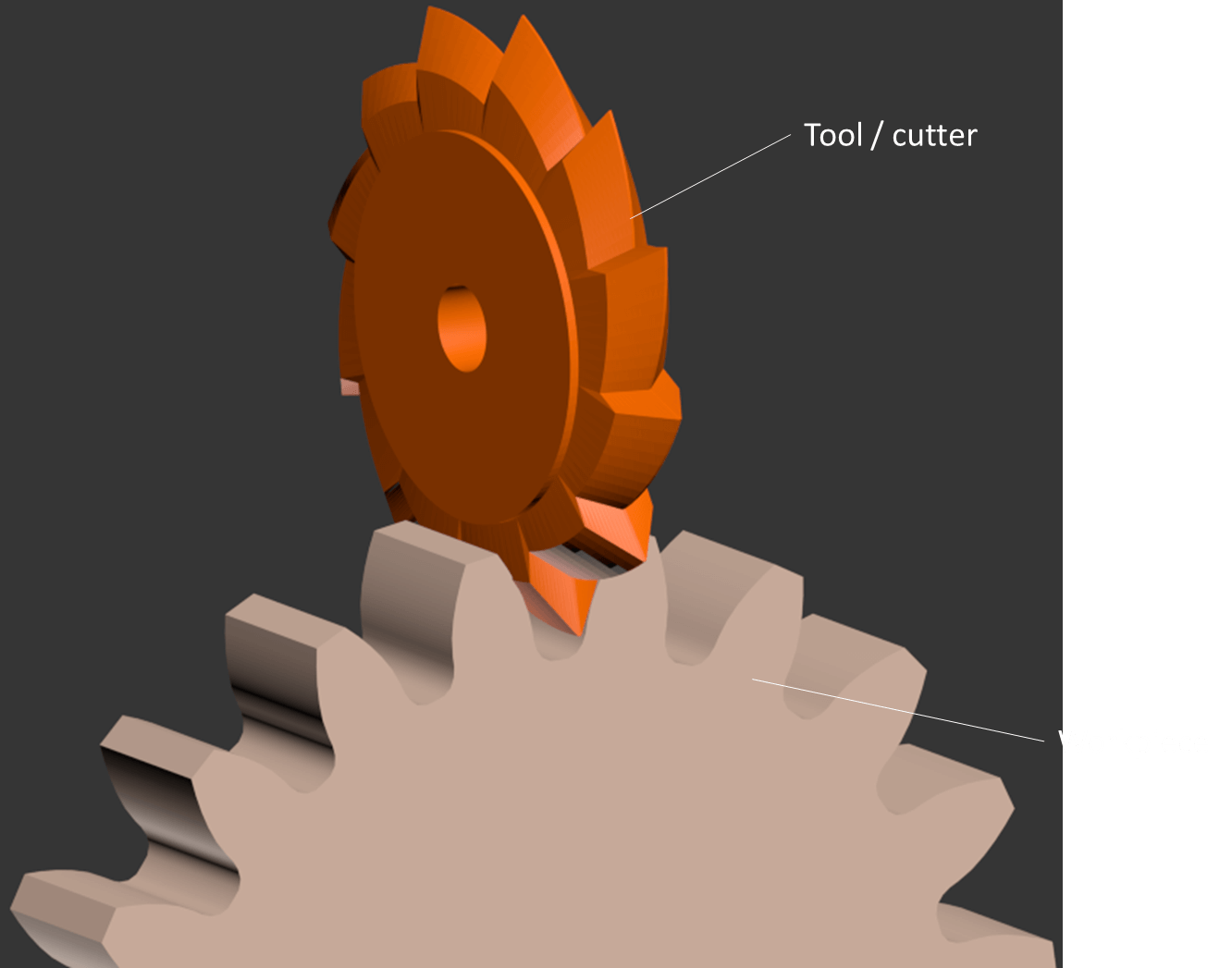
Milling gears is slow because it creates extensive heat transfer to the workpiece, which means that successive teeth should not be milled one right after the other.
Electrical discharge Machining (EDM)
EDM is an electromechanical manufacturing process where material is removed from a workpiece by applying a series of current discharges between two electrodes separated by a dielectric bath liquid. Rather than cutting, the spark acts as a ‘cutting tool’ that actually erodes the material instead.
EDM is good at cutting complex geometries of all sizes, even as a gear cutting process, but the process has its limitations. If you don’t have good control and precise programming, it’s easy to damage part surfaces — especially curved teeth profiles that are challenging for CNC programs to execute. But high-quality and intuitive 3D modeling and CAM software — like Feature CAM, Autodesk Fusion, Master CAM, and others — can produce the smooth motion needed to cut curved teeth.
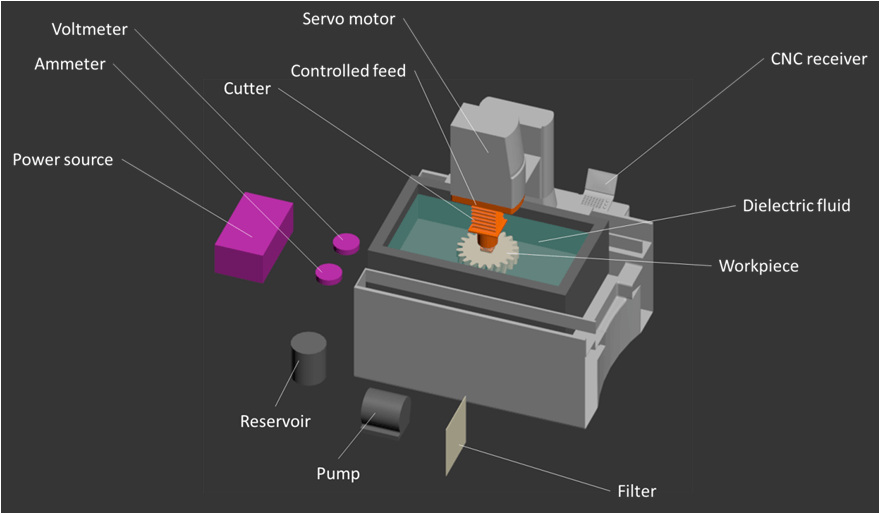
EDM machines have improved in recent years, which has minimized issues with surface finishes, and improved cutting precision and resulting material properties (microstructure, mechanical properties, etc). The process can achieve tight tolerances as small as thousandths of an inch and create both small (diameter of fractions of an inch) and large gears (diameter of over 20 inches). This process is used for both delicate applications in watches and clocks and to cut more robust gears like those used in race cars.
Gear Forming
Rolling
Rolling is one of the oldest gear forming processes that hot or cold rolls a blank workpiece through two or three dies, as seen below.
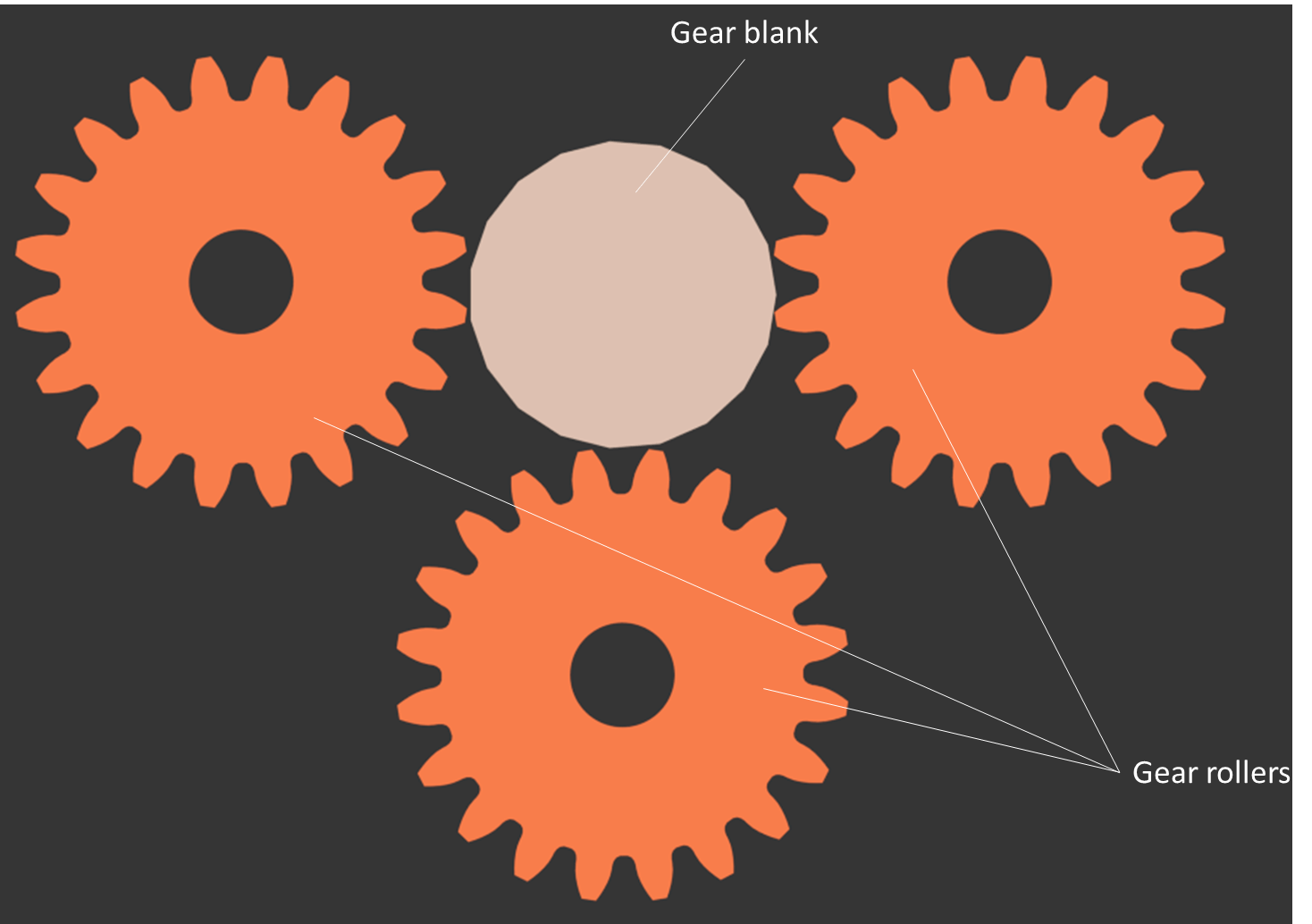
When material saving is a critical concern during manufacturing, rolling is a good option since there’s no chip generation. However, to get an efficient process, you must consider rolling parameters, deformations, and microstructure effects before ramping up production.
Casting
Casting is a forming process whereby molten metal is poured into a mold cavity to form shapes. Gear casting is used to create gear blanks (that are then machined) and full gears with cast teeth profiles. Tolerances and accuracy are crucial considerations when casting gears, and creating casting molds entails a lot of up-front costs. However, once the mold and process parameters are determined, large production quantities justify the investment.
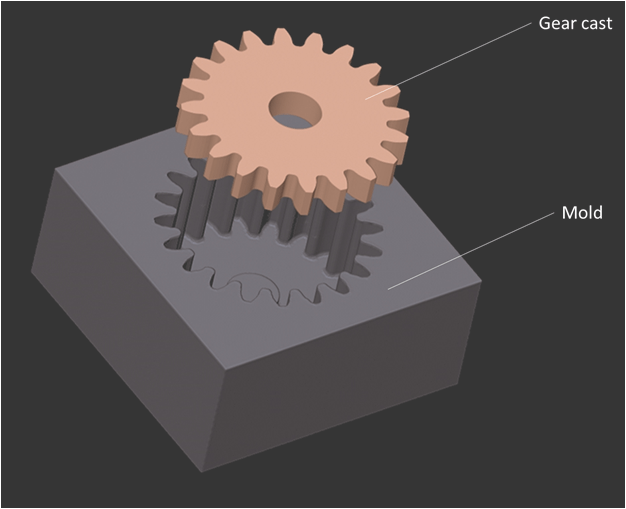
Sand casting is used primarily to produce gear blanks to be used in other processes. Fully functioning spur, helical worm, cluster, and bevel gears are all made by gear casting and are used in washing machines, small appliances, hand tools, toys, and cameras.
Powder Metallurgy
Powder metallurgy is a high-precision forming method that’s a cost-effective alternative to conventional, machine-finished steel and cast iron gears. However, this method is not suited for larger gear sizes but is adept at creating small, high-quality spur, bevel, and spiral gears. Because of the porosity of the formed material, larger gears have less fatigue and impact resistance, though a sintering process can be used to improve their mechanical properties.
Powder metallurgy is also particularly useful when gear designs include holes, depressions, and different surface levels or projections. You’ll find these gears in appliances, farm, lawn, and garden equipment, automobiles, trucks, and military vehicles.
Additive Manufacturing
Also known as 3D printing, additive manufacturing constructs a three-dimensional object, layer by layer, from a CAD 3D model. Due to the nature of the process, additive machines can form complex designs with lattice structures modeled to achieve mass reduction not easily obtained through conventional methods. This type of geometry is often created using 3D topology optimization and generative computer design.
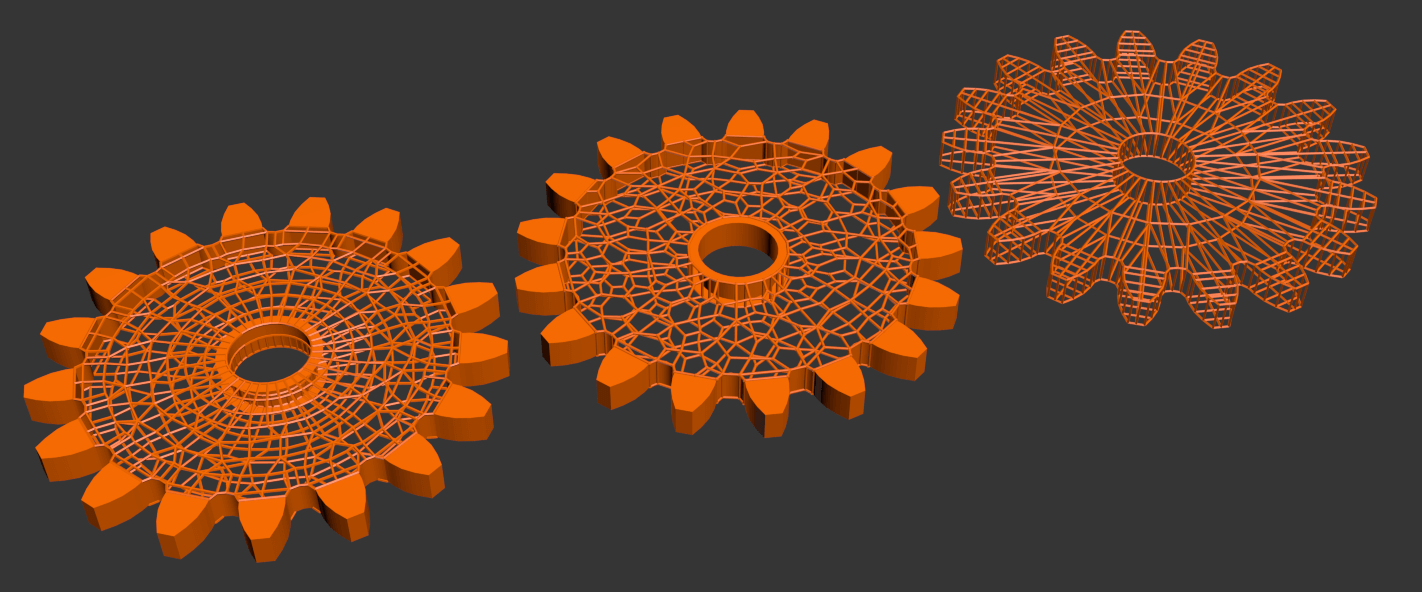
Conventional and non-circular gears can be fabricated with additive manufacturing processes, and high-quality 3D printers are relatively affordable and widely available. Because of this availability, it’s become the choice for repairs and mechanical projects like educational toys or other gadgets needing fully functional gears. You can also include additional features and even combine geometry with the gear shapes to add customized shafts, keys, or grooves to the same solid.
So, now you know more of the many options for maching gears, whether you’re generating, forming, or cutting them. For help with all your CNC design projects, including gear design, check out our helpful eBook, CNC Design Guide.
Sourcing Simplified – Start Your Next Project With Fictiv
No matter what sort of gears you need, Fictiv can fabricate them.
We’re experts at producing custom, precision gears through our CNC machining services in a variety of materials, and we simplify custom part sourcing with intelligent, streamlined, automated workflows. Fictiv is your operating system for custom manufacturing that makes gear and mechanical part procurement faster, easier, and more efficient. Create an account and upload your part to see what our instant quote process, design for manufacturability feedback, and intelligent platform can do for you.